●Quantum effects in the brain
- 人工進化研究所(AERI)
- May 19, 2023
- 4 min read
Quantum Artificial Intelligence:
Consciousness-driven bio-computer
with quantum interference-controlled BMIVLSI implemented in cranial nerves
Physicist Brain Scientist Visiting Professor of Physics,
California Institute of Technology
Ph.D. & Dr. Kazusho Kamuro
AERI:Artificial EvolutionResearch Institute
1200 East California Boulevard Pasadena, California 91125
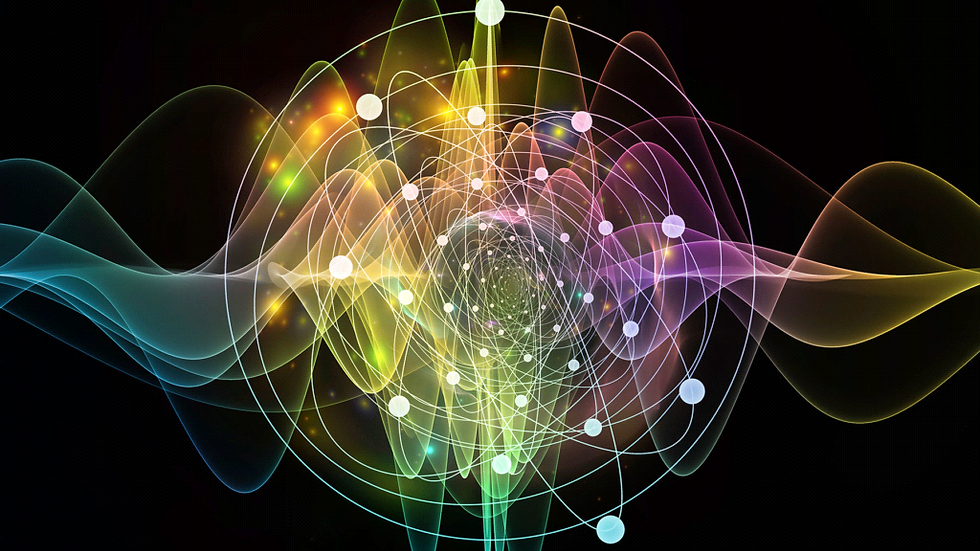
●Quantum effects in the brain
While the weirdness of quantum theory has lent itself to some unhelpful pseudoscientific interpretations of consciousness, there has been resistance from scientists to yoke the two together.
Just because both subjects are difficult to understand, does not mean that they necessarily inform each other. Despite this, the first detailed theory of quantum consciousness emerged in the 1990s from the Nobel-prize winning University of Oxford physicist Roger Penrose and anaesthesiologist Stuart Hameroff from the University of Arizona (Mathematics and Computers in Simulation 40 453).
Their “orchestrated objective reduction” (Orch OR) theory has undergone a number of revisions since its inception (Physics of Life Reviews 11 39), but generally it posits that quantum computations in cellular structures known as microtubules have an effect on the firing of neurons and, by extension, consciousness.
The theory elicited a number of criticisms but perhaps the most damning followed from the fundamental tenets of quantum theory. A quantum system – which might refer for example to the dynamics of a photon – is a delicate thing. Conventionally, quantum effects are observed at low temperatures where this system is isolated from destructive interactions with its surrounding environment. This would seem to exempt quantum effects from playing any role in the mess and fuss of living systems. Biological systems, such as the brain, operate at physiological temperatures and are unavoidably bound to their environments. As calculated by physicist Max Tegmark at Princeton University in 2000, quantum effects would not survive long enough to have any influence on the much slower rates at which neurons fire (Phys. Rev. E 61 4194).
However, this objection has to some extent been mitigated by research done in the broader field of quantum biology. The application of quantum theory in a biological context has had most success with regards to photosynthesis but research on the avian compass, olfaction, enzymes and even DNA also suggest that quantum effects might be implicated more generally in the functioning of biological organisms.
In a trivial sense all biology is quantum mechanical just as all matter is quantum mechanical – it is made up of atoms and thus subject to the physical laws of atomic structure first formalized by Bohr at the beginning of the 20th century. The focus of quantum biology, however, is on key quantum effects – those quantum phenomena that seem to defy our classical imaginations, such as superposition states, coherence, tunnelling and entanglement (see box “Quantum phenomena”).
If this is the what of quantum effects in the brain, the where is more straightforward. The brain is made up of nerve cells – elongated cells consisting of a cell body, dendrites and axon (figure 1). Put simplistically, information is passed to and from the brain by the firing or not firing of neurons, a process determined by a nerve cell’s electrochemical potential. This potential depends on the spread of charged ions across the cell membrane, making either side of the membrane more or less positive. In order for a nerve to fire, its resting potential must be increased to the requisite threshold potential. How this signal then passes from one cell to the next is still a matter of debate, but the accepted theory is that this neural communication is managed by chemicals known as neurotransmitters released into the synaptic cleft, which then bind to receptors of the next nerve cell, thereby altering its electrochemical gradient and causing neural activation.
Figure1
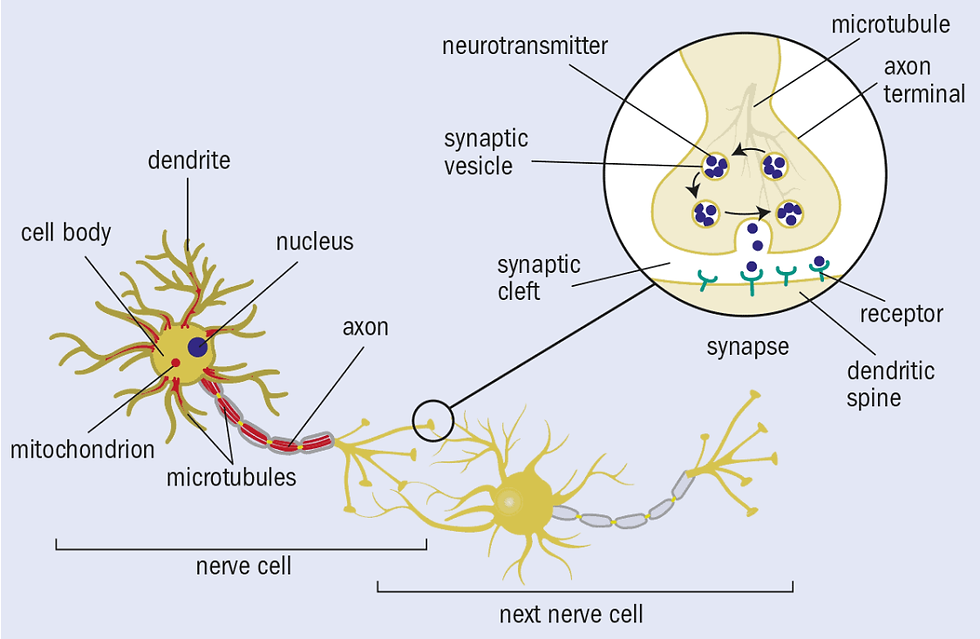
Figure1 shows 1 The structure and function of a nerve cell. Quantum effects in the brain might be better phrased as quantum effects in neural processes, for which this diagram of a nerve cell serves as illustration. Nerve cells consist of three main elements – the cell body, which contains the various organelles; dendrites, which receive incoming signals; and the axon, which transmits this signal. It is thought that signals are passed between nerves where the axon terminal of one nerve cell meets the dendritic spines of the next, at the synaptic cleft. As a signal moves through a nerve cell and reaches the axon terminal, it triggers the release of neurotransmitters into the synaptic cleft.
Neurotransmitters bind to receptors on the neural membrane of dendritic spines, opening ion channels and thus altering the next cell’s membrane potential, passing along the signal.
Nerve constituents that are important to a discussion of quantum effects are the microtubules, which are formed from the polymerization of a protein known as tubulin, and the mitochondria, often described as the energy centres of the cell. Microtubules give structure to the cellular cytoskeleton and are necessary for cell division as well as the movement of motor proteins, a group of proteins that convert chemical to mechanical energy. The mitochondria use electron transport chains and proton gradients to create adenosine triphosphate (ATP), which powers biological processes. They are also the proposed primary site of biophoton production. (Illustration by Angela Illing. Reproduced from AVS Quantum Sci. 2 022901, with the permission of the American Vacuum Society)
Comments