The Singular Power Generation Approach Terawatt Scale Offering the Sole 1 Cent per kWh CHEGPG system
- 人工進化研究所(AERI)
- Sep 21, 2023
- 11 min read
Professor Kamuro's near-future science predictions:
The Singular Power Generation Approach
at the Terawatt Scale
Offering the Sole 1 Cent per kWh:
CHEGPG system
Quantum Physicist and Brain Scientist
Visiting Professor of Quantum Physics,
California Institute of Technology
IEEE-USA Fellow
American Physical Society-USA Fellow
PhD. & Dr. Kazuto Kamuro
AERI:Artificial Evolution Research Institute
Pasadena, California
✼••┈┈••✼••┈┈••✼••┈┈••✼••┈┈••✼••┈┈••✼••┈┈••✼
1. Household Electricity Tariffs by Country
Below, we present household electricity tariffs by country in Table 1.
The residential electricity tariffs in our country are approximately twice as high as those in the United States.
Table 1. Household Electricity Tariffs by Country

2. Country-Specific Industrial Electricity Tariffs
Table 2 presents the country-specific industrial electricity tariffs.
Table2.
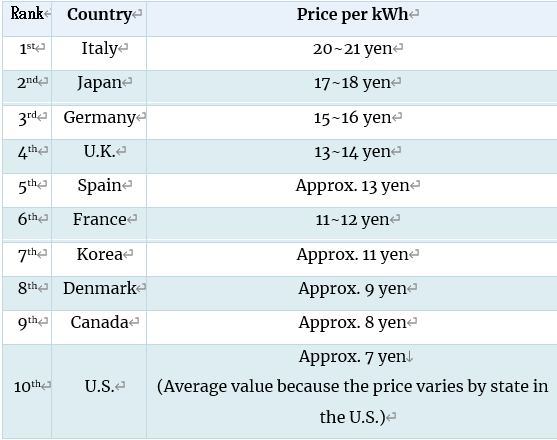
The industrial electricity tariffs in our country are the second highest in the world, making them exceptionally expensive.
3. Country-Specific Residential Electricity Tariffs
Table 3 illustrates the historical trends in country-specific residential electricity tariffs.
In our country, the rate was approximately 25 yen per kWh until 2015.
Table3
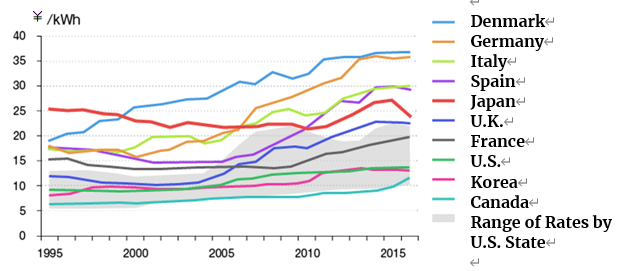
4. Cost per 1 kWh of Electricity Generation by Generation Method in 2021
The costs associated with coal-fired and LNG-fired power generation tend to be higher when factoring in carbon mitigation expenses and fuel price increases. Additionally, renewable energy sources such as wind and solar power face challenges related to grid stability, contributing to higher costs. Nuclear power generation, on the other hand, exhibits relative cost stability due to its lower dependence on fuel costs. However, it is important to acknowledge the presence of social costs stemming from the risk of accidents.
5. Cost Projections per 1 kWh of Electricity Generation by Generation Method (Estimated for 2030)
The cost projections for constructing and operating new power generation facilities in a greenfield setting by the year 2030 have been officially released by the national government.
a. Oil-fired power generation, with an assumed operational lifespan of 40 years, is projected to cost approximately 24.9 yen or more per 1 kWh.
b. Natural gas-fired power generation, with an assumed operational lifespan of 40 years, is projected to cost approximately 10.7 yen or more per 1 kWh.
c. Coal-fired power generation, with an assumed operational lifespan of 40 years, is projected to cost approximately 13.6 yen or more per 1 kWh.
d. Solar power generation, with an assumed operational lifespan of 25 years, is projected to cost approximately 8.2 yen or more per 1 kWh.
e. Nuclear power generation, with an assumed operational lifespan of 40 years, is projected to cost approximately 11.7 yen or more per 1 kWh.
f. Onshore wind power generation, with an assumed operational lifespan of 25 years, is projected to cost approximately 9.8 yen or more per 1 kWh, while offshore wind power generation is projected to cost approximately 25.9 yen or more per 1 kWh.
g. Geothermal power generation, with an assumed operational lifespan of 40 years, is projected to cost approximately 16.7 yen or more per 1 kWh.
h. Hydropower generation, with an assumed operational lifespan of 40 years, is projected to cost approximately 10.9 yen or more per 1 kWh.
i. Biomass power generation, with an assumed operational lifespan of 40 years, is projected to cost approximately 13.2 yen or more per 1 kWh.
j. Cogeneration power generation, with an assumed operational lifespan of 30 years, is projected to cost approximately 9.3 yen or more per 1 kWh.
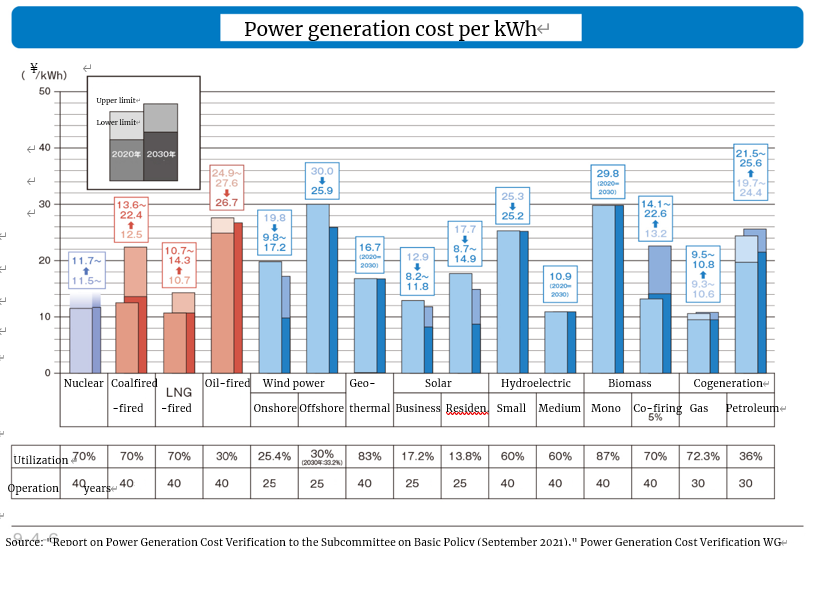
6. The Lifespan of Steam Turbines in Thermal Power Generation
The Lifespan of Steam Turbines in Thermal Power Plants is influenced by a multitude of factors. Typically, it spans several decades, contingent upon specific equipment and operational conditions.
The following are the principal factors that impact the lifespan of steam turbines:
a. Maintenance and Maintenance Practices: Whether appropriate maintenance and regular upkeep are conducted significantly affects the turbine's lifespan. Proper maintenance can potentially extend its operational life.
b. Fuel Type and Operating Conditions: The lifespan of a turbine varies depending on the type of fuel it uses and the operating conditions. Generally, high-temperature, high-pressure steam turbines can generate electricity efficiently, but they may experience wear and corrosion in high-temperature environments, potentially reducing their lifespan.
c. Design and Manufacturing Quality: The design and manufacturing quality of the turbine have a substantial influence on its lifespan. The use of high-quality materials and manufacturing processes can contribute to extending its lifespan.
d. Technological Advancements: The energy industry is a rapidly evolving field, and the introduction of new technologies and improved designs can serve as a means to extend the lifespan of older turbines through upgrades and enhancements.
e. Environmental Regulations: Changes or stringency in environmental regulations can impact the operational conditions and lifespan of thermal power plants. Modifications may be necessary to comply with new regulations.
Generally, the lifespan of steam turbines can range from 20 to over 50 years, depending on specific facilities and operational conditions. Owners and operators of thermal power plants strive to maximize the turbine's lifespan through proper maintenance, refurbishment, and, if necessary, upgrading to newer technologies and equipment.
7. The Lifespan of Wind Turbine Power Generation Systems
Wind turbines, also known as wind power generators, have a finite lifespan influenced by various factors. Typically, the lifespan of a wind turbine is estimated to be around 20 to 25 years. The following are the primary factors that affect the lifespan of wind turbines:
a. Maintenance and Maintenance Practices: Whether appropriate maintenance and regular upkeep are conducted significantly impacts the lifespan of a wind turbine. Components of the wind turbine, especially rotating parts and the generator, require routine inspection and repair.
b. Wind Conditions: The wind conditions at the location where the wind turbine is installed affect its lifespan. High wind speeds and consistent wind patterns can enhance the turbine's efficiency. However, in cases of extremely high wind speeds, the turbine may experience increased stress, potentially shortening its operational life.
c. Technological Advancements: Wind power technology is a rapidly evolving field, with new wind turbine designs and materials constantly being developed. Older turbines may be less efficient and reliable compared to newer technologies, prompting consideration of upgrades or replacements to extend their lifespan.
d. Environmental Factors: Environmental factors, weather conditions, salt corrosion (in the case of turbines near coastal areas), frost, snowfall, and more can also influence the lifespan of wind turbines.
e. Operational Conditions: The operational load and uptime during operation also impact the lifespan of wind turbines. Continuous high loads or operation under harsh conditions can increase stress on the turbine.
Owners and operators of wind turbines take measures such as proper maintenance, inspections, and upgrades to maximize the lifespan of their turbines. Additionally, when a wind turbine reaches the end of its lifespan, it needs to be dismantled and undergo recycling or proper disposal. Efforts toward sustainable waste management of wind turbines are ongoing within the wind power industry.
8. Lifespan of Solar Photovoltaic Systems
Solar photovoltaic (PV) systems, commonly referred to as solar panels, exhibit a finite lifespan influenced significantly by the quality of their design and manufacturing. Generally, the longevity of solar photovoltaic systems is determined by the following factors:
a. Panel Quality: The quality of solar panels has a substantial impact on their lifespan. High-quality panels tend to degrade slowly, maintaining high efficiency over extended periods. Conversely, low-quality panels degrade prematurely, leading to decreased efficiency.
b. Maintenance and Upkeep: Whether proper maintenance and regular cleaning are conducted significantly affects the lifespan of solar photovoltaic systems. Factors such as dirt, dust, and shading can influence panels and potentially reduce their efficiency.
c. Environmental Factors: Solar panels are installed outdoors and are exposed to various environmental factors such as wind, rain, snow, ultraviolet radiation, and temperature fluctuations. The durability of solar panels can be influenced by these environmental conditions.
d. Inverters and Electronic Components: Solar photovoltaic systems include electronic components like inverters. The lifespan of these components also needs to be considered. In general, inverters may have a shorter lifespan compared to solar panels.
The typical lifespan of solar photovoltaic systems is estimated to be in the range of 25 to 30 years. However, with high-quality panels and proper maintenance, it is possible to extend their lifespan. Additionally, introducing new panels and components in line with technological advancements can potentially prolong the system's lifespan.
When the lifespan of solar photovoltaic panels approaches its end, recycling and waste disposal considerations come into play to minimize environmental impact. Efforts are underway in the industry to promote sustainable waste management practices for end-of-life solar panels.
9. Lifespan of Thermal Power Plants
The lifespan of thermal power plants is significantly dependent on a multitude of factors, including the type of power plant, equipment type, operational conditions, maintenance practices, and refurbishments. Generally, thermal power plants can have lifespans extending several decades, yet the factors influencing this longevity are diverse:
a. Fuel Type: Thermal power plants utilize various fuel types, including coal-fired power plants, natural gas-fired power plants, and oil-fired power plants, each with distinct lifespans. Coal-fired power plants, for example, may experience shorter lifespans due to environmental concerns and technological advancements.
b. Equipment Type: Thermal power plants employ a variety of equipment and technologies for electricity generation. These encompass conventional steam turbines, gas turbines, combined-cycle power plants, and emerging high-efficiency power plants, each characterized by varying lifespans.
c. Maintenance and Upkeep: The execution of proper maintenance and routine upkeep profoundly impacts the lifespan of thermal power plants. Regular inspections, repairs, and equipment upgrades contribute to extending the operational life.
d. Technological Advancements: The energy industry stands as a rapidly progressing sector, marked by technological advancements such as efficient power generation and emissions reduction technologies. Consequently, older thermal power plants may become inefficient and experience shorter lifespans.
e. Environmental Regulations: Alterations or heightened stringency in environmental regulations can affect operational conditions and lifespans of thermal power plants. Facility modifications may be necessitated to comply with new regulations.
Typically, the lifespan of thermal power plants ranges from 20 to over 50 years. To extend this lifespan, it is imperative to implement proper maintenance practices, adapt to evolving environmental regulations, incorporate new technologies, and optimize operational conditions. Additionally, decommissioning and waste disposal considerations are commonly addressed when the lifespan of a thermal power plant concludes.
10. The Lifespan of Turbines in Different Types of Thermal Power Plants
The lifespan of turbines in various types of thermal power plants varies based on several factors. Thermal power plants encompass a range of technologies, including traditional steam turbines, gas turbines, combined-cycle power plants, and emerging high-efficiency power plants. Each type of power plant exhibits distinct characteristics that influence the lifespan of its turbines. Here, we delve into the turbine lifespans for each category:
a. Traditional Steam Turbine Power Plants:
Traditional steam turbine power plants generally have a relatively long lifespan.
They can typically operate for more than 30 years, and with proper maintenance and upgrades, their lifespan can be extended.
b. Gas Turbine Power Plants:
Gas turbine power plants often have shorter lifespans compared to steam turbines.
A typical lifespan ranges from 20 to 30 years, although facility improvements and maintenance may extend it.
c. Combined-Cycle Power Plants:
Combined-cycle power plants are highly efficient systems that combine gas and steam turbines.
These plants usually have lifespans exceeding 30 years and have the potential to provide high efficiency and low emissions.
d. Emerging High-Efficiency Power Plants:
Emerging high-efficiency power plants utilize state-of-the-art technologies to achieve high efficiency and low environmental impact.
A lifespan of over 30 years is expected, but advancements in technology may necessitate upgrades.
These estimated lifespan guidelines are general in nature, and actual power plants' lifespans can vary due to factors such as fuel type, equipment quality, operational conditions, maintenance practices, and regional regulations. Owners and operators of thermal power plants typically employ sound management and operation practices to ensure an optimal lifespan. Additionally, they may consider facility upgrades or replacements as necessary to meet evolving industry standards and technological advancements.
11. The Lifespan and Generation Cost per 1 kWh of the Closed-Cycle Heat Exchange Power Generation System with Regenerative Binary Engines (CHEGPG system)
a. CHEGPG system, currently under research and development by the Artificial Evolution Research Institute (AERI https://www.aeri-japan.com/ ), represents a groundbreaking advancement in power generation technology. This innovative system efficiently harnesses low-temperature binary gas (resembling steam) to drive power turbines. In stark contrast to conventional thermal power generation methods, CHEGPG system eliminates the use of coal or gas combustion, thereby avoiding the associated issues of reduced operational lifespan. Additionally, it boasts a zero-emission profile, effectively mitigating the release of greenhouse gases such as CO2. As a result, with proper corrosion management and maintenance, it holds the potential for an operational lifespan exceeding 100 years, all while delivering exceptional efficiency and maintaining a zero environmental footprint.
b. CHEGPG system power plants achieve remarkable cost efficiency through the following key characteristics:
Utilization of Geothermal Energy (1): These power plants harness geothermal energy as their primary source, significantly reducing reliance on external energy resources.
Regeneration of Binary Gas (2): By effectively regenerating binary gas, the system maximizes energy utilization and cost efficiency.
Extended Lifespan of Power Generation Equipment (3): With an operational lifespan exceeding 100 years, effectively approaching infinity, the cost of electricity generation is maintained at less than 1 yen per 1 kWh.
Furthermore, ongoing optimization of binary gas and enhancements to heat recovery mechanisms hold the theoretical potential to further reduce the cost of electricity generation to as low as 0.1 to 0.01 yen per 1 kWh.
These attributes collectively establish CHEGPG system power plants as highly cost-effective, sustainable, and adaptable solutions for electricity generation, with the capacity for substantial cost reductions through continuous improvements in binary gas optimization and heat recovery mechanisms.
c. CHEGPG system distinguishes itself in several key ways from traditional power generation methods that rely heavily on imports of coal, oil, or LPG:
100% Dependence on Local Geothermal Resources (1): Unlike conventional power generation methods that rely on imports for nearly 100% of their energy sources, CHEGPG system utilizes locally sourced geothermal energy.
Stable Terawatt-Level Generation Throughout the Year (2): In contrast to solar and wind power generation methods, CHEGPG system can achieve a stable terawatt-level electricity generation capacity throughout the entire year.
Significant Reduction in Generation Costs (3): Current power generation methods typically require generation costs ranging from 8.2 yen to 25.9 yen per 1 kWh. In comparison, CHEGPG system has the potential to reduce generation costs to less than 1 yen per 1 kWh (theoretically as low as 0.1 to 0.01 yen).
These unique characteristics position CHEGPG system as a transformative solution that achieves energy self-sufficiency through local geothermal resources, ensures year-round stable terawatt-level electricity generation, and significantly lowers electricity generation costs compared to conventional methods.
d. Due to the reasons mentioned above, it is considered that CHEGPG system, under active research and development by the General Incorporated Association Artificial Evolution Research Institute (AERI: https://www.aeri-japan.com/ ), belongs to the most promising group capable of ensuring our nation's energy security.
e. In addition to the reasons mentioned above, it can be understood that only the Closed-Cycle Heat Exchange Power Generation System with Binary Engine Incorporation (CHEGPG system), which holds the potential of generating 1,000 terawatts (1 terawatt = 1 trillion watts = 1,000 MW) per unit (a single generation method), can simultaneously address the transformation challenges and critical issues of our nation's energy security, (1) guaranteeing the aforementioned energy security, (2) mitigating climate change and addressing climate-related issues, (3) enabling Green Transformation (GX), (4) achieving carbon neutrality, and not only that, (5) achieving zero emissions of greenhouse gases like CO2, (6) fostering the creation and development of Green Giants in the power generation sector, and (7) creating and developing industries based on emission trading through carbon pricing, all of which currently lack viable solutions or approaches.
END
**************************************************************************
Quantum Brain Chipset & Bio Processor (BioVLSI)
Prof. PhD. Dr. Kamuro
Quantum Physicist and Brain Scientist involved in Caltech & AERI Associate Professor and Brain Scientist in Artificial Evolution Research Institute( AERI: https://www.aeri-japan.com/ )
IEEE-USA Fellow
American Physical Society Fellow
PhD. & Dr. Kazuto Kamuro
email: info@aeri-japan.com
--------------------------------------------
【Keywords】 Artificial Evolution Research Institute:AERI
HP: https://www.aeri-japan.com/
#ArtificialBrain #ArtificialIntelligence #QuantumSemiconductor #Quantumphysics #brain implant-type biocomputer #BrainScience #QuantumComputer #AI #NeuralConnectionDevice #QuantumInterference #QuantumArtificialIntelligence #GeoThermalpoAERIr #MissileDefense #MissileIntercept #NuclearDeterrence #QuantumBrain #DomesticResiliency #Quantumphysics #Biologyphysics #Brain-MachineInterface #BMI #BCI #nanosizeSemiconductors #UltraLSI #nextgenerationSemiconductors #opticalSemiconductors #NonDestructiveTesting #LifePrediction #UltrashortpulseLasers #UltrahighpoAERIrLasers #SatelliteOptoelectronics #RemoteSensing #GeoThermalpoAERIr #RegenerativeEnergy #GlobalWarming #CimateCange #GreenhouseGses #Defense #EnemystrikeCapability #QuantumBrain #QuantumBrain #QuantumArtificialIntelligence #ArtificialBrain #QuantumInterference #cerebralnerves #nextgenerationDefense #DefenseEectronics #Defense #RenewableEergy #LongerInfraStructurelife #MEGAEarthquakePrediction #TerroristDeterrence #NonDestructivetesting #LifespanPrediction #ExplosiveDetection #TerroristDetection #EplosiveDetection #VolcaniceruptionPrediction #EnemybaseAtackCpability #ICBMInterception #RemoteSensing #BioResourceGowthEnvironmentAssessment #VolcanicTremorDetection #volcanicEruptiongGasDetection #GreenhousegasDetection #GlobalWarmingPrevention #ArtificialIntelligence #BrainScience #AI #MissileDefense #MissileInterception #NuclearAERIaponsdisablement #Nuclearbaseattack #DefensiveAERIapons #eruptionPrediction #EarthquakePrediction #QuantumBrain #QuantumConsciousness #QuantumMind #QuntumBrain #QuntumBrainComputing #QuntumBrainComputer #AtificialBrain #ArtificialIntelligence #BrainComputing #QuantumBrainChipset #BioProcessor #BrainChip #BrainProcessor #QuantumBrainChip #QuantumBioProcessor #QuantumBioChip #brain-computer #brain implant-type biocomputer #BrainInplant #Reprogrammable #self-assembly #MolecularComputer #MolecularBrain implant-type biocomputer #military #BrainImplant #militaryhardware #militaryweapon #unmannedweapon #combataircraft #robotarmor #militaryweapon #cyborg #soldier #armor #strategicweapon #combatKilling #AntiNuclearwarfare #roboticweapons #weaponsindustry #weaponofmassdestruction #MilitarySoldier #RobotSOLDIER #BrainImplant #chemicalWarefare #chemicalBattlefield #WarEconomic #HumanitarianStrategy #NextGenerationWarfare #BiologicalWarefare #BiologicalBattlefield #EnemyBaseAttackAbility
#brain #implant-type #biocomputer #BrainInplant #Reprogrammable #selfassembly #MolecularComputer #MolecularBrain #implant-type #biocomputer # #military #BrainImplant #militaryhardware #militaryweapon #unmannedweapon #combataircraft #robotarmor #militaryweapon #cyborg #soldier #armor #strategicweapon #combatKilling #AntiNuclearwarfare #roboticweapons #weaponsindustry #weaponofmassdestruction #MilitarySoldier #RobotSOLDIER # #BrainImplant #chemicalWarefare #chemicalBattlefield #WarEconomic #HumanitarianStrategy #NextGenerationWarfare #BiologicalWarefare #BiologicalBattlefield #EnemyBaseAttackAbility #LaserDefenseSystem #HAMIIS #PetawattLaser #HexaWattLaser #UltraHighPowerLaser #ChirpedPulseAmplification #CPA #OpticalParametricAmplification #OPA #HighEnergyPhysics #Defense #Security #MissileDefenseSystem #LaserInducedPlasma #Supernovae #Pulsar #Blackhole #FemtosecondLaser #CavityDumping #ModeLocking #FemtosecondPulse #LaserSpectroscopy #UltrafastSpectroscopy #MultiphotonMicroscopy #NonlinearOptics #FrequencyConversion #HarmonicHGeneration #ParametricAmplification #MaterialProcessing #Micromachining #SurfaceStructuring #LaserAblation #MultiphotoMicroscopy #Ophthalmology #LAM #LandAttackMissiles #ASWM #AntiSubmarineWarfareMissiles
Comments